The stability of scale factor and zero bias of Q-Flex accelerometers is crucial in assessing the performance of accelerometers. During the measurement of minute motion acceleration, certain physical factors such as external magnetic interference and fluctuating temperature fields can affect the stability of scale factor and zero bias of accelerometers.
This article addresses the issue of magnetic field and temperature variations affecting the stability of scale factor and zero bias of quartz flexible accelerometers, discussing the adoption of rigorous electromagnetic shielding structures and temperature control structures to enhance the measurement accuracy of acceleration devices.
1.Working Principle of Accelerometer
The acceleration measurement device adopts the principle of quartz flexible accelerometer measurement, using four quartz flexible accelerometers as sensors in a redundant design of four axes. Among them, two accelerometers measure vertical acceleration along the Z-axis and -Z-axis (improving measurement accuracy through data fusion), while the other two measure horizontal acceleration along the X-axis and Y-axis.
By improving the internal structure of the accelerometer device, rigorous electromagnetic shielding and precise temperature control are employed to enhance measurement accuracy and achieve high-precision three-dimensional motion acceleration measurement of the carrier.
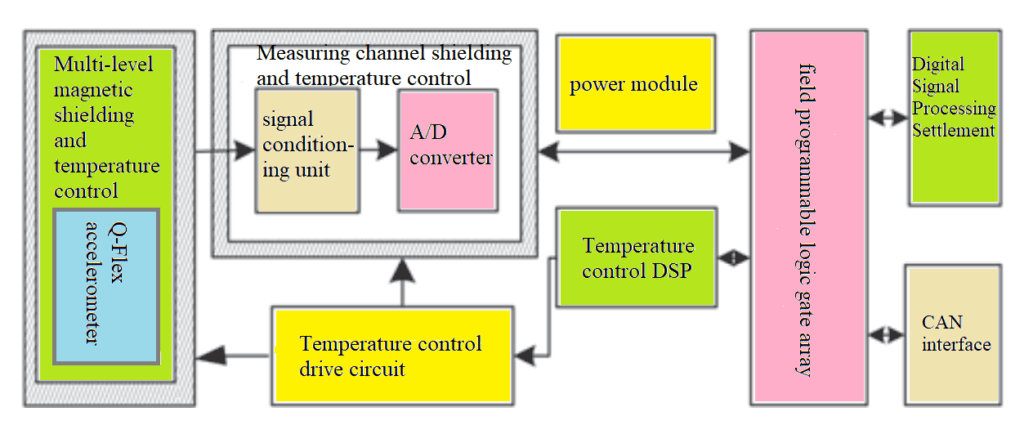
2.Structural Design of Quartz flexible Accelerometer Measurement Device
The accelerometer measurement device adopts a frame assembly structure with heat sinks installed around the shell, a base installed at the bottom, connected to the mounting platform, and electrical connectors set at the top for information communication.
The accelerometer measurement device mainly consists of a frame, quartz flexible accelerometers, shielding structures for accelerometers and measurement channels, temperature control structures for accelerometers and measurement channels, and accelerometer mounting structures. The accelerometer mounting structure is composed of a rectangular block of aviation aluminum with four cylinders carved out. This mounting structure ensures the fixed positions of each accelerometer and reduces the overall device volume. The structure of the accelerometer measurement device is shown in Fig. 2.
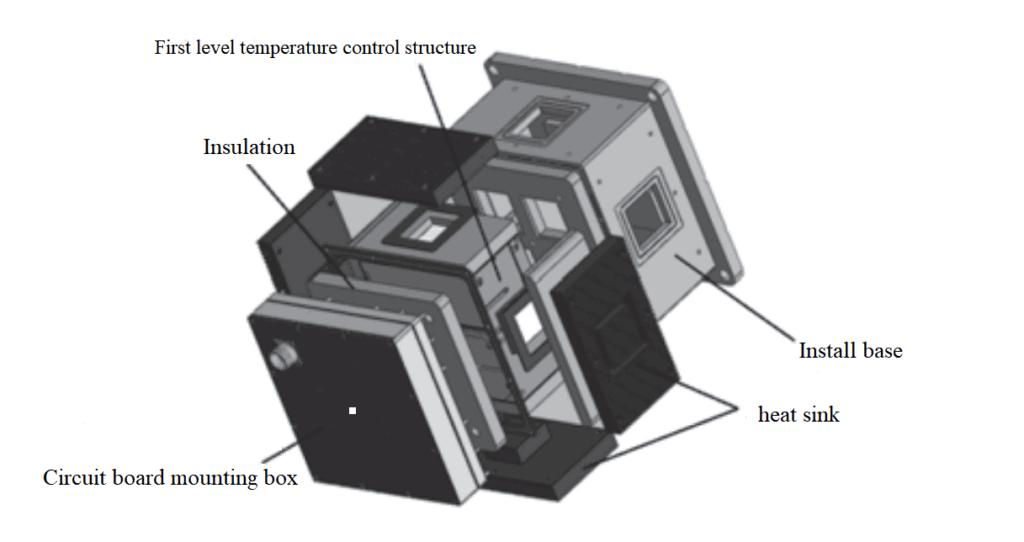
2.1 Shielding Structure Design
2.1.1 Accelerometer Shielding Structure Design
External magnetic fields can cause lag and non-linear effects on the scale factor of quartz flexible accelerometers. Experimental data suggests that a magnetic field variation of 10Gs can cause a scale factor change of 5~20ppm.
Considering the inevitable openings and seams in the accelerometer measurement device, which may cause magnetic leakage, the magnitude of magnetic leakage mainly depends on the maximum linear dimension of the openings. Therefore, the shielding structure of the accelerometer measurement device adopts as many small-area openings as possible to replace large-area openings to enhance the shielding effect. Furthermore, the shielding material selected is the Permalloy alloy 1J85, which has a high permeability and effectively suppresses low-frequency magnetic field interference.
Considering the reflection, absorption, and other factors of the shielding structure, the overall shielding effect can be represented as follows:
Where A is the absorption loss, R is the reflection loss, Bs is the correction term caused by multiple reflections inside the shielding layer, x is the thickness of the shielding layer, μr is the relative permeability, σr is the relative conductivity, f is the frequency of the interfering magnetic field, and δ is the skin depth.
From the above, it can be seen that for low-frequency magnetic field interference, the shielding effect of Permalloy alloy is excellent. However, this material has a low magnetic saturation intensity, and it is easy for Permalloy alloy to reach magnetic saturation when encountering higher magnetic field intensity. Therefore, the accelerometer measurement device adopts a dual-layer shielding structure to reduce magnetic leakage.
The first layer of shielding uses aviation aluminum, which has a high magnetic saturation intensity and low permeability; the second layer of shielding uses Permalloy alloy, which has a high permeability. After passing through the first layer of shielding, the external interfering magnetic field is significantly attenuated, and then, using the high permeability of the second layer of shielding, the interfering magnetic field can be further reduced to a very low level. The decomposition view of the accelerometer shielding structure is shown in Fig. 4.
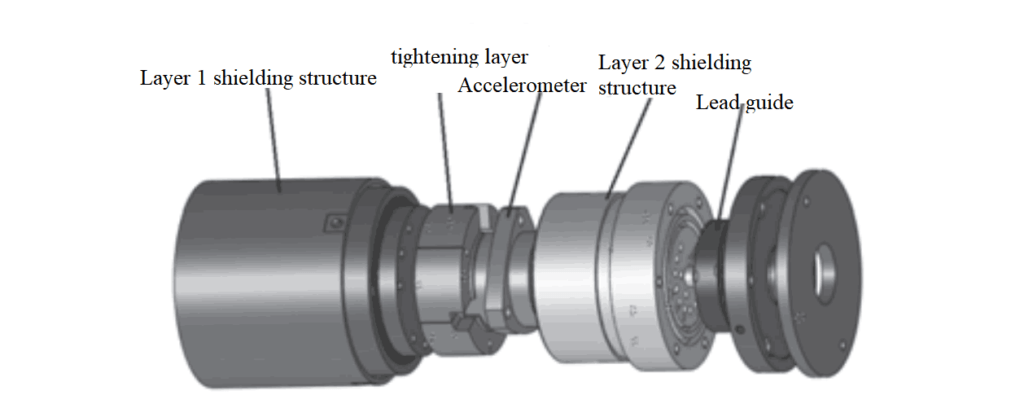
2.1.2 Measurement Channel Shielding Structure Design
The shielding structure of the measurement channel consists of an upper cover and a lower cover assembled into a shielding shell, made of Permalloy alloy 1J85. Each channel is wrapped in a shielding shell to achieve electromagnetic shielding of the measurement channel. The shielding structure of the measurement channel is shown in Fig. 5.
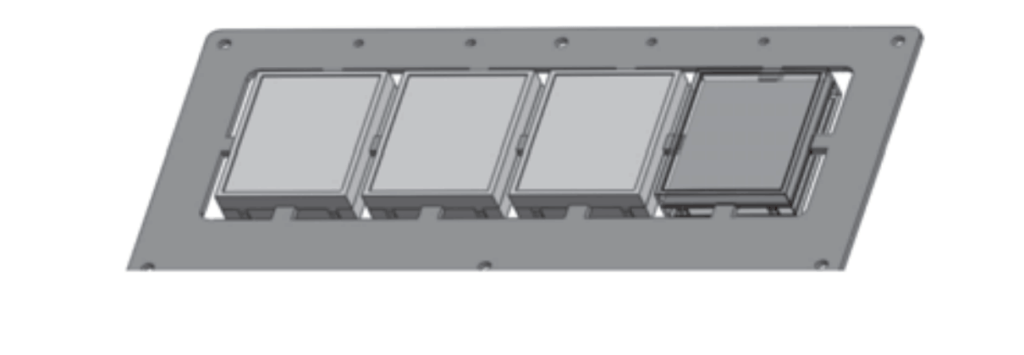
2.2 Temperature Control Structure Design
2.2.1 Accelerometer Temperature Control Structure Design
Temperature changes can affect the zero bias and scale factor of quartz flexible accelerometers. There are two main factors causing temperature changes in accelerometers: first, after the device starts, the internal temperature of the device will gradually rise, causing the temperature of the accelerometer to change; second, the torque coil inside the device will heat up when powered, causing the temperature of the accelerometer to change.
The working environment temperature of the accelerometer measurement device varies greatly, while the accelerometer needs to meet the working environment requirement of 0°C to 50°C, making precise temperature control very challenging. Conventional single-stage temperature control schemes are difficult to meet the temperature control requirements.
To achieve an accuracy of ±0.1°C in accelerometer temperature control, the accelerometer measurement device adopts a two-stage precise temperature control structure. The two-stage temperature control structure is shown in the decomposition in Fig. 6. The temperature stability accuracy of the first-stage temperature control structure is designed to be ±0.5°C, with a temperature control target of 30°C.
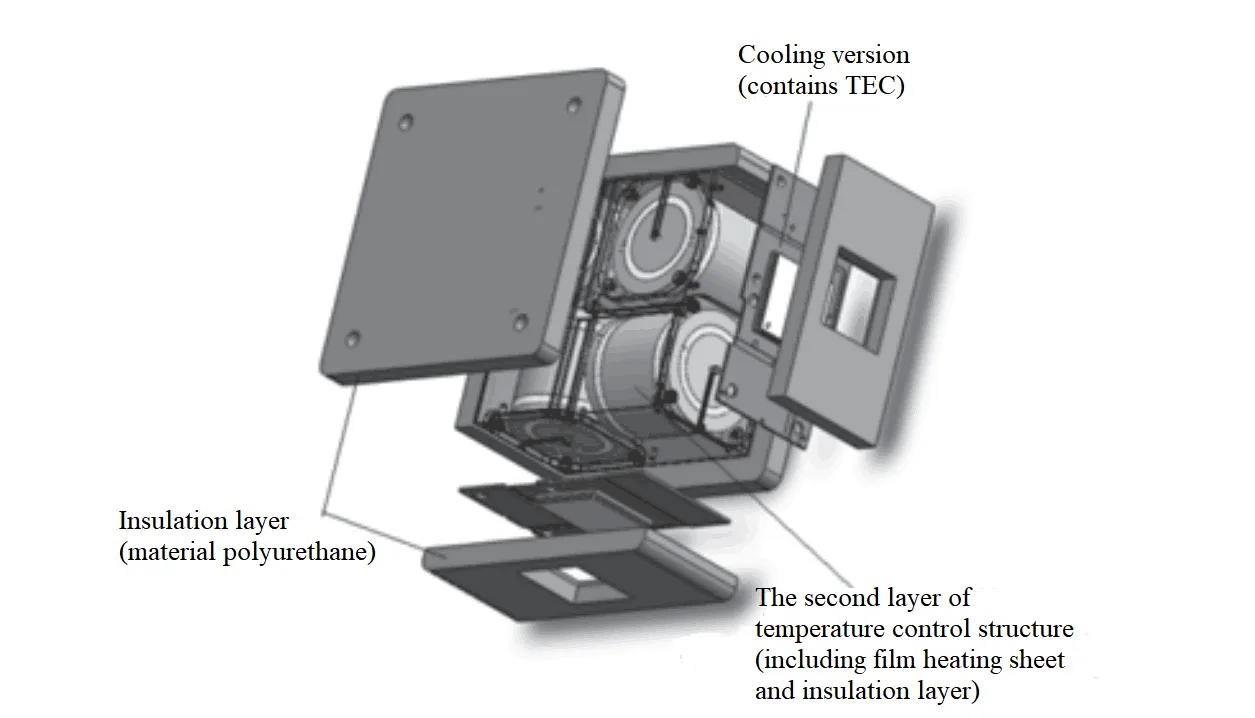
A digital temperature sensor DS18B20 is used as the temperature measurement sensor, and a thermoelectric cooler (TEC) is used as the temperature control component. Changing the direction and magnitude of the control current can achieve different heating or cooling powers using the thermoelectric cooler.
The temperature accuracy of the second-stage temperature control structure is designed to be ±0.1°C, matching the target accuracy of the accelerometer, with a temperature control target of 45°C, maintaining a certain temperature difference with the first-stage temperature control environment. The second-stage temperature control structure uses platinum resistance as the temperature measurement sensor, with stable temperature coefficients. Thin film heaters are used as the temperature control components, wrapped around the first layer of shielding of the accelerometer, achieving different heating powers by changing the control current.
To improve temperature control stability, the accelerometer is insulated with polyurethane as the insulation material, which has low thermal conductivity and good processing performance, effectively achieving thermal insulation.
2.2.2 Measurement Channel Temperature Control Structure Design
The temperature control structure of the measurement channel is similar to the first-stage temperature control structure of the accelerometer, using a thermoelectric cooler (TEC) for cooling or heating. One side of the TEC is closely attached to the upper and lower covers of the shielding shell of the measurement channel, and the other side is closely attached to the heat sink housing. The shielding shell is wrapped with insulation, made of polyurethane. The temperature control structure of the measurement channel is shown in Fig. 7.
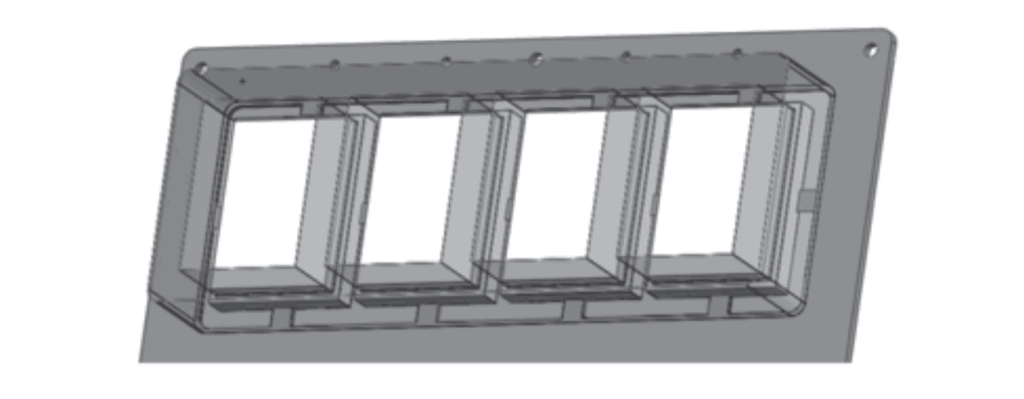
Conclusion
This paper proposes an accuracy improvement scheme for the influence of external magnetic fields and temperature variations on the measurement accuracy of quartz flexible accelerometers. By optimizing the structure and designing accelerometer magnetic shielding structures and precise two-stage temperature control structures, the stability of scale factor and zero bias is enhanced, thereby improving measurement accuracy.
It is worth noting that the high-performance quartz flexible accelerometer ER-QA-03A has an effective vibration acceleration of 6 grms, using high-quality quartz crystals, capable of adapting to high-temperature, high-pressure, high-vibration environments, and various environmental conditions with high acceleration applications, exhibiting extremely high reliability and stability.
In addition, the ER-QA-03A1 series of accelerometers have a scale factor of 15 ppm and bias repeatability of 10 μg, achieving high-precision measurements. Apart from extensive applications in aerospace and military fields, it is also widely used in automotive, medical, and research fields. In scientific research, it can be used for studying earthquakes, crustal movements, cosmology, and microgravity environments.
More Technical Questions
1. In-Depth Exploration: What Exactly is Satellite Timing?
2. Methods to Maintain the Long-Term Performance of Quartz Flexure Accelerometers
3. Analysis of Temperature Characteristics of Quartz Flexible Accelerometer
4. MEMS Accelerometers for Analyzing Mechanical Vibrations
5. Maximizing GNSS Applications in Land Vehicles Systems
6. Failure Mode Analysis of Quartz Flexure Accelerometer
Products in Article
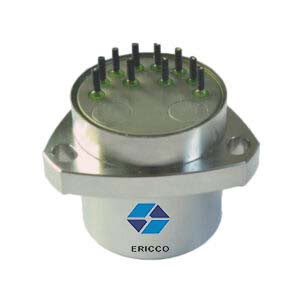
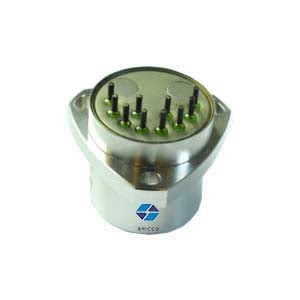
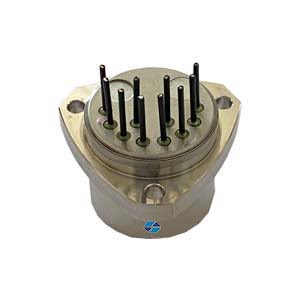
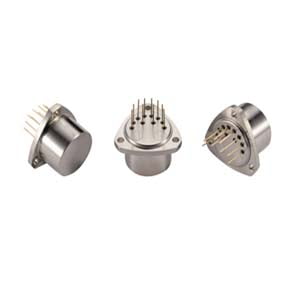
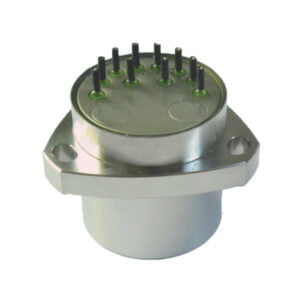
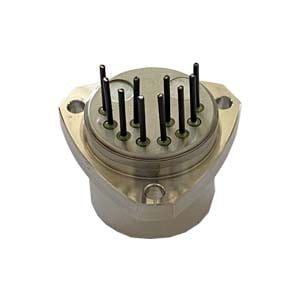